Investigations into Velocity and Distance Perception Based on Different Types of Moving Sound Sources with Respect to Auditory Virtual Environments
DOI:
https://doi.org/10.20385/1860-2037/10.2013.4Keywords:
Auditory Scene Analysis, Auralization, Doppler Shift, Moving Sound Source, Spatial HearingAbstract
The characteristics of moving sound sources have strong implications on the listener's distance perception and the estimation of velocity. Modifications of the typical sound emissions as they are currently occurring due to the tendency towards electromobility have an impact on the pedestrian's safety in road traffic. Thus, investigations of the relevant cues for velocity and distance perception of moving sound sources are not only of interest for the psychoacoustic community, but also for several applications, like e.g. virtual reality, noise pollution and safety aspects of road traffic. This article describes a series of psychoacoustic experiments in this field. Dichotic and diotic stimuli of a set of real-life recordings taken from a passing passenger car and a motorcycle were presented to test subjects who in turn were asked to determine the velocity of the object and its minimal distance from the listener. The results of these psychoacoustic experiments show that the estimated velocity is strongly linked to the object's distance. Furthermore, it could be shown that binaural cues contribute significantly to the perception of velocity. In a further experiment, it was shown that - independently of the type of the vehicle - the main parameter for distance determination is the maximum sound pressure level at the listener's position. The article suggests a system architecture for the adequate consideration of moving sound sources in virtual auditory environments. Virtual environments can thus be used to investigate the influence of new vehicle powertrain concepts and the related sound emissions of these vehicles on the pedestrians' ability to estimate the distance and velocity of moving objects.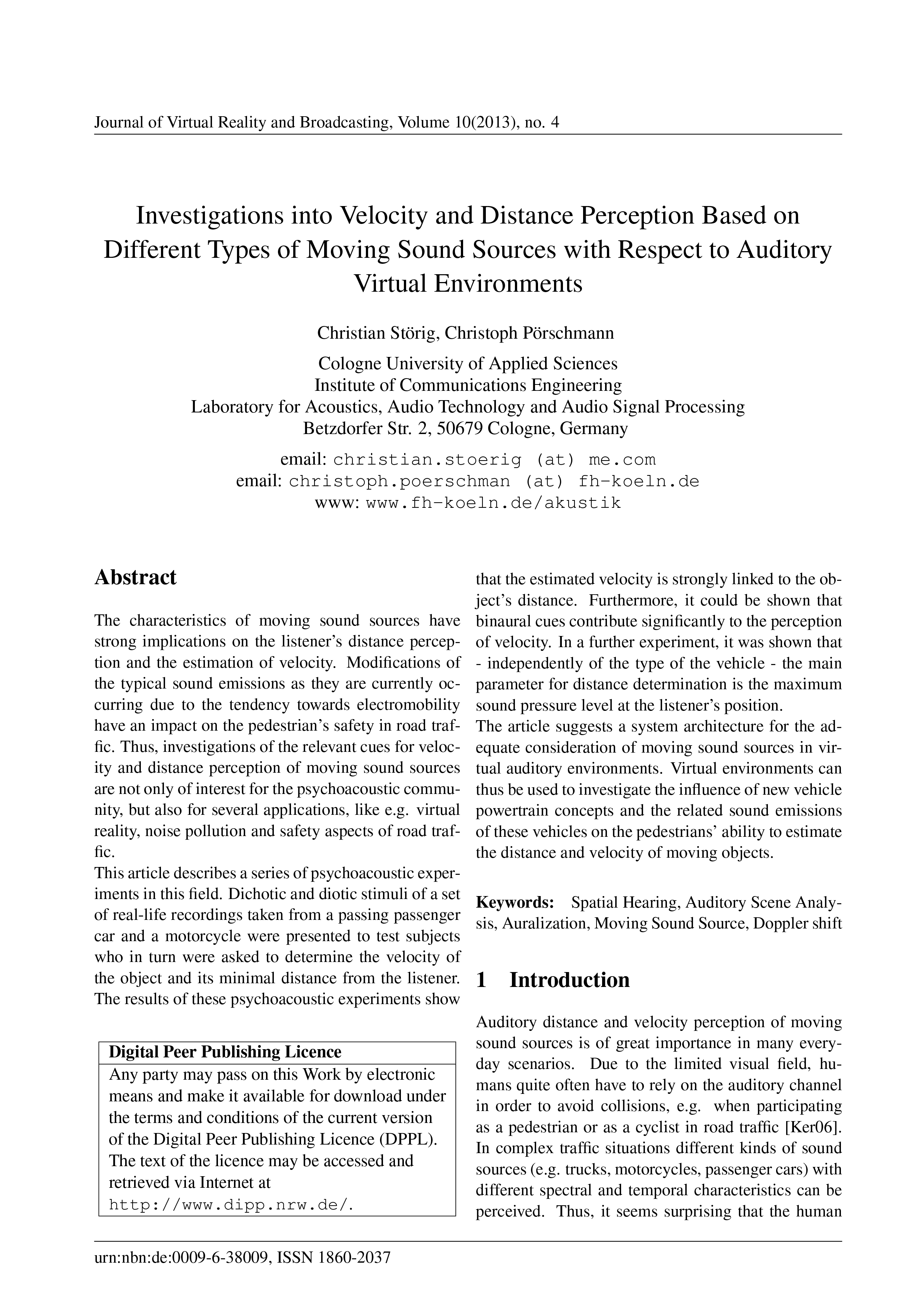
Published
2014-01-14
Issue
Section
Articles